- Waste management in Japan
- Circular economy in Japan
- Waste management in Asia
- Disaster waste management
Energy-Saving Wastewater Treatment with Anaerobic Technology
Introduction
Anaerobic wastewater treatment is a biological process of decomposing organic contaminants in human and industrial effluents by metabolic activities of microorganisms under anaerobic condition without oxygen to ensure protection of human health and the environment. Previously, our online magazine introduced the application of methane fermentation technology to the treatment of wet biomass, such as food wastes ("Household Biogas Production System in Rural Asia - A Case Study in China" in the May 2012 issue). The anaerobic technology has also been applied to the treatment of wastewater as energy-saving technology. Recently in Japan, anaerobic technology has been widely adopted by the food, beverage, chemical and paper industries for treating their industrial effluent.
Anaerobic wastewater treatment has technical requirements that are slightly different from the above-mentioned methane fermentation of wet biomass. Such requirements have prompted various unique research and development efforts. This article explains the technical characteristics of anaerobic wastewater treatment and the progress made in its research and development.
Aerobic and anaerobic wastewater treatment
Household and industrial wastewater treatment uses a sequence of physical, chemical and biological processes. Among them, biological unit process represents the core part of the treatment of organic contaminants. The role of the unit process is to have microorganisms decompose the organic matter dissolved in the wastewater. Anaerobic and aerobic systems are two types of biological wastewater treatment depending on the types of microorganisms that they use. In Japan, aerobic system is used at almost all domestic wastewater treatment facilities including sewage treatment plants, Johkasou (household treatment tanks used in Japan), and industrial wastewater treatment plants (IWWTPs) for specific facilities discharging wastewater that may cause harm to human health and the environment. On the other hand, only about one percent of IWWTPs, except for small-scale ones, have adopted relatively new anaerobic systems. However, the number of IWWTPs that have replaced their aerobic reactors with anaerobic ones has been increasing every year.
Aerobic treatment is a process of decomposing organic contaminants in wastewater by metabolic activities of microorganisms under aerobic condition with sufficient oxygen. The chemical equation below approximates the reaction of aerobic microorganisms decomposing the organic matter.

As shown above, the decomposition of organic matter by aerobic microorganisms is a process of converting organic matter into CO2 and microbial cells with consumption of a large amount of oxygen.
In contrast, the reaction of anaerobic microorganisms is typically expressed by the equation below although there are other types of anaerobic decomposition which may not generate methane gas.

As shown above, the decomposition of pollutants by anaerobic microorganisms is a process of converting organic matter into methane and CO2. Like aerobic microorganisms, anaerobic microorganisms also convert organic matter into microbial cells. However, it accounts for only a small percentage, and therefore it is generally omitted from the equation.
Advantages and disadvantages of anaerobic treatment
Table 1 shows the characteristics of anaerobic treatment in comparison with aerobic treatment. First, anaerobic treatment has the following advantages. As shown in Equation (1), aerobic decomposition requires the volume of dissolved oxygen that is proportional to the volume of organic matter in the wastewater. The oxygen concentration in the biological reaction tank needs to be constantly and properly controlled. The electricity required to supply sufficient dissolved oxygen for treating 1 kg of COD (Chemical Oxygen Demand: a measurement unit for organic matter) is 1.1 kWh, which accounts for most of the energy consumption for kg COD removal for wastewater treatment facilities. On the other hand, anaerobic treatment does not require oxygen, and therefore does not require electricity for supplying oxygen. Although both treatments use electricity for stirring and pumping operations, these operations require very little power. Thus, anaerobic decomposition is more energy-saving and relatively easier to control. In addition, as mentioned earlier, microbial cell synthesis always occurs in biological wastewater treatment process, and if any excess cells are produced, they are regarded as waste (excess sludge). The amount (dry weight) of excess sludge generation from 1 kg COD removal is 0.4 to 0.6 kg with aerobic treatment, and 0.03 to 0.15 kg with anaerobic treatment. Therefore, the fact that anaerobic treatment generates much smaller amount of waste, offers another advantage. Furthermore, as shown in Equation (2), anaerobic treatment generates methane gas, that is, energy. Methanation of 1 kg COD can produce about 3,300 kcal of energy.
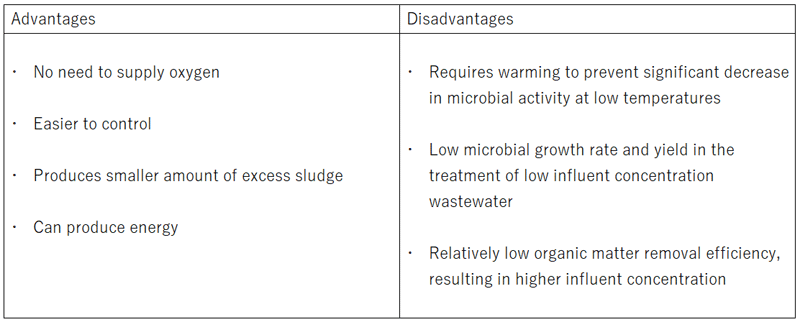
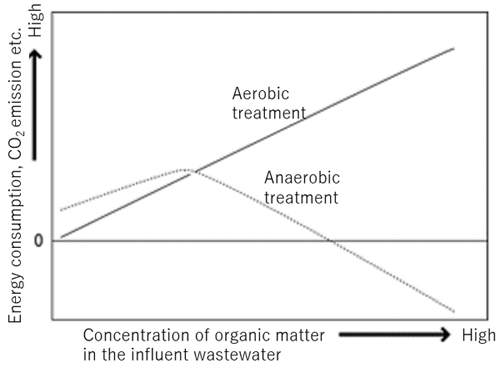
Conversely, the following three points are the main disadvantages of anaerobic treatment: (1) it requires energy to warm reaction tanks ("warming energy"), (2) low microbial growth rate, and (3) relatively low organic matter removal efficiency. Anaerobic treatment requires the warming of reactors in order to maintain microbial activity. The warming energy occupies most of the energy consumption for anaerobic treatment. The difference between the amount of methane production and the warming energy per volume of wastewater is the net energy recovery. If the difference becomes negative, anaerobic treatment will be considered an energy consuming process, similar to aerobic treatment. My past article in this magazine (see the December 20, 2010 issue: Is Bio-gasification Technology an Energy Producing Process or Energy Consuming Process? [in Japanese]) elaborated this point using an energy balance model. The same article explained that the concentration of organic matter in theinfluent is the key factor to determine whether the energy balance is positive or negative. It also governs the relative merits of aerobic and anaerobic treatments. Fig.1 is a conceptual diagram drawn from the analytical results of Cakir and Stenstrom.1 As shown in the diagram, aerobic treatment consumes less energy when the concentration of organic matter is low in the influent. However, when the concentration reaches a certain level, the use of anaerobic treatment becomes more beneficial. In this way, this important diagram helps us when selecting either of the two technologies, but this topic has not yet been fully studied, and more research is needed. Another disadvantage of anaerobic treatment is that it has low conversion yield of organic matter to microbial cells, although this item was already listed as its advantage (see Table 1). Anaerobic treatment requires higher influent concentration to achieve reasonable concentration of microorganisms retained in the reaction tank. When the influent concentration is above a certain level, the concentration of anaerobic microorganisms in the reaction tank becomes saturated. On the other hand, when the influent concentration is low, the reaction tank cannot retain a sufficient amount of microorganisms to ensure satisfactory performance because of their low growth yield. In addition, organic matter removal efficiency in anaerobic treatment is slightly lower than that in aerobic treatment because affinity of substrate is lower for anaerobic microorganisms than for aerobic ones.2 In other words, anaerobic microorganisms cannot efficiently uptake low concentration of organic matter compared to aerobic ones, which result in higher influent concentration. Over the last several decades, overcoming such disadvantages has been the biggest challenge for the research and development of anaerobic treatment.
Research and development to overcome disadvantages
What is needed to overcome the above-mentioned three disadvantages of anaerobic treatment (the low reaction rate at low temperature, the low microbial growth rate, and the low organic matter removal efficiency)? Past research and development results have provided some answers: (1) making retention time for the microorganisms longer than that for the wastewater in reaction tanks (to overcome the first and second disadvantages), (2) adopting a reaction tank that combines anaerobic treatment with simple aerobic treatment or separation membranes (to overcome the third disadvantage). In Japan, the research and development efforts for (1) began in the 1960s. Its first application, so-called anaerobic contact process, combined an anaerobic reaction tank and a sedimentation tank with recycling. The precipitated microorganisms in the sedimentation tank were returned into the reaction tank to concentrate retained microorganisms there. In the 1980s, reaction tanks using either fluidized or fixed biological beds were researched extensively. These tanks used carrier media to which anaerobic microorganisms are affixed to be grown in order to keep their concentration high inside. In the 1980s and 1990s, a new anaerobic treatment method, called UASB (Up-flow Anaerobic Sludge Blanket) method, was developed by utilizing a phenomenon of forming well settleable microbial aggregate (microbial granules) in an up-flow reactor with a reverse funnel-shaped gas-liquid-solid separator. Having succeeded in making the reactors retain these granules of concentrated microorganisms (each has a diameter of a few millimeters), the UASB method and more advanced EGSB (Expanded Granular Sludge Bed) method contributed to the most significant and remarkable practical application of anaerobic technology in the industrial wastewater treatment facilities worldwide. Most anaerobic reactors in the industrial wastewater treatment facilities in Japan also use these methods.3 For example, all industrial breweries in Japan have adopted this method. Regardless of the influent concentration, this method enables high concentration of microorganisms in anaerobic reactors, many times higher than that of aerobic treatment. As a result, the reactor achieves higher reaction rate, which can make up for the effect of decreased microbial activity at low temperatures. In addition to the above, another method installing separation membranes in reaction tanks was developed to achieve (2). This newer method allows treated wastewater to pass through the membranes to be drawn off afterwards. The application of this method to the actual facilities began in the 2000s and has been expanded since then. This newer method also provides the function of (1) as microbial cells do not permeate the membranes.
These research and development results have made anaerobic technology applicable for the treatment of much wider range of wastewater. However, practical application of anaerobic technology started only after aerobic treatment had become widespread. Therefore, anaerobic technology still has a great potential for application in Japan, and its successful installation has been found only in the treatment of specific types of wastewater which represents only a small part of the entire wastewater in the country. In order to make anaerobic technology more mature, some operational and administrative issues still need to be solved. Meanwhile, its possible applications have recently been explored in some specific fields. They include domestic wastewater treatment in development countries where costly aerobic technology has not yet been widespread, and industrial wastewater treatment which has also had difficulties in adopting aerobic technology. In this way, continuous efforts have been made to promote and establish anaerobic treatment technology.
References
- Cakir, F.Y., Stenstrom, M.K. (2005) Greenhouse gas production: A comparison between aerobic and anaerobic wastewater treatment technology. Water Research, 39, 4197-4203
- Kobayashi T., Kanazawa T., Yasui H., Li Y.Y. (2012) Comparison of self-degradation characteristics of waste activated sludge between aerobic and anaerobic conditions. Journal of Japan Sewage Works Association, 49 (600), 107-114
- Kobayashi T., Yan F., Takahashi S., Li Y.Y. (2011) Effect of starch addition on the biological conversion and microbial community in a methanol-fed UASB reactor during long-term continuous operation. Bioresource Technology, 102, 7713-7719